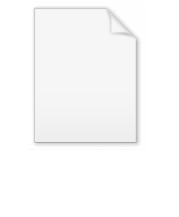
Strangeness production
Encyclopedia
Strangeness production is a signature and a diagnostic tool of quark-gluon plasma
(or QGP) formation and properties. Unlike up
and down quark
s, from which everyday matter is made, strange quark
s are formed in pair production
processes in collisions between constituents of the plasma. The dominant mechanism of production involves gluon
s only present when matter has changed into the QGP phase. When QGP disassembles into hadron
s in a breakup process, the high availability of strange antiquarks
helps to produce antimatter containing multiple strange quarks, which is otherwise rarely made. Similar considerations are at present made for the heavier charm quark
flavor which is made earlier on at the beginning of the collision process in first interactions and is only abundant in the high-energy environments that will be provided by CERN
's Large Hadron Collider
.
in the universe
is found in atomic nuclei
, which are made of neutron
s and proton
s. These neutrons and protons are made up of smaller particles called quarks. For every type of matter particle
there is a corresponding antiparticle
with the same mass and the opposite charge. It is hypothesized that during the first few instants of the universe
, it was composed of almost equal amounts of matter and antimatter, and thus contained nearly equal number of quarks and antiquarks. Once the Universe expanded and cooled to a critical temperature
of approximately , quarks combined into normal matter and antimatter. Antimatter annihilated
with matter up to the small initial asymmetry of about one part in five billion, leaving the matter around us. Free and separate individual quarks and antiquarks have never been observed in experiments—quarks and antiquarks are always found in groups of three (baryon
s), or bound in quark–antiquark pairs (meson
s).
s after the Big Bang, in a very hot gas
of free quarks, antiquarks and gluon
s. This gas is called a quark–gluon plasma (QGP), since the quark-interaction charge (color charge
) is mobile and quarks and gluons move around. This is possible because at a high temperature the early universe is in a different vacuum state, in which normal matter can not exist but quarks and gluons can, they are deconfined
. In order to recreate this deconfined
phase of matter
in the laboratory it is necessary to exceed a minimum temperature or, equivalently, energy density
. Nuclear collisions
at relativistic energies are the experimental tool that has been developed in the past 30 years to form, study, and explore quark–gluon plasma in laboratory. In a small space domain we thus create for a very short time conditions akin in its properties to the early Universe at the age of 10–40 microseconds. Discovery of this new QGP state of matter
has been announced both at CERN
and at Brookhaven National Laboratory
(BNL). At this time comprehensive experimental evidence about its properties is being assembled.
The process of the formation of quark–gluon plasma lasts little longer than the time the light takes to pass through the volume occupied by the atomic nucleus used to produce the ultra-high pressure and temperature in the highly-energetic collision. After this brief time the hot drop of quark plasma evaporates in a process called hadronization
. The short duration of the laboratory's collision illustrated above makes the study of free quarks in quark–gluon plasma an experimental challenge.
and Hagedorn
. Unlike the up and down quarks, strange quarks are not brought into the reaction by the colliding nuclei. Therefore, any strange quarks or antiquarks observed in experiments have been "freshly" made from the kinetic energy of colliding nuclei. Conveniently, the mass
of strange quarks and antiquarks is equivalent to the temperature or energy at which protons, neutrons and other hadron
s dissolve into quarks. This means that the abundance of strange quarks is sensitive to the conditions, structure and dynamics of the deconfined matter phase, and if their number is large it can be assumed that deconfinement conditions were reached.
s: gluons are the wavy lines; strange quarks are the solid lines; time runs from left to right. The bottom section is the process where the heavier quark pair arises from the lighter pair of quarks shown as dashed lines. The gluon fusion process occurs almost ten times faster than the quark based strangeness process, and allows achievement of the high thermal yield where the quark based process would fail to do so during the duration of the "micro-bang". The gluon collisions here are occurring within the thermal matter phase and are thus different from the high energy processes that can ensue in the early stages of the collisions when the nuclei crash into each other. The heavier, charm and bottom quarks are produced there dominantly. The study in relativistic nuclear (heavy ion) collisions of charmed and soon also bottom hadronic particle production beside strangeness will provide complementary and important confirmation of the mechanisms of formation, evolution and hadronization of quark gluon plasma.
Strange quarks also bind with the heavier charm and bottom quarks which also like to bind with each other. Thus in presence of a large number of these quarks quite unusually abundant exotic particles can be produced, some of these have never been observed before. This should be the case in the forthcoming exploration at the new Large Hadron Collider
at CERN of the particles that have both charm and strange quarks, and even bottom quarks as components.
and decay by weak interaction
s into lighter quarks on a timescale that is extremely long compared with the nuclear-collision times. This makes it relatively easy to detect strange particles through the tracks left by their decay products. Consider as example the decay of a negatively charged Xi baryon (green in figure, dss), into a negative pion
(d) and a neutral Lambda baryon (uds). Subsequently, the Lambda decays into a proton and another negative pion. In general this is the signature of the decay of a Xi. Although the negative Omega baryon (sss) has a similar final state decay topology, it can be clearly distinguished from the Xi because its decay products are different.
Measurement of abundant formation of Xi (uss/dss), Omega (sss) and especially their antiparticles is an important cornerstone of the claim that quark-gluon plasma has been formed. This abundant formation is often presented in comparison with the scaled expectation from normal proton-proton collisions; however, such a comparison is not a necessary step in view of the large absolute yields which defy conventional model expectations. The overall yield of strangeness is also larger than expected if the new form of matter has been achieved. However, considering that the light quarks are also produced in gluon fusion processes, one expects increased production of all hadron
s. The study of the relative yields of strange and non strange particles provides information about the competition of these processes and thus the reaction mechanism of particle production.
, France
These results on Antilambda formation in S-S reaction shown in the figure indicate a significant enhancement of the production of this antimatter particle comprising one antistrange quark as well as antiup and antidown quarks. All three constituents of the Lambda particle are newly produced in the reaction. The expected no-quark-gluon plasma production yield is shown at the bottom of the figure. These results are presented as function of the variable called rapidity
which characterizes the speed of the source. The peak of emission indicates that the additionally formed antimatter particles do not originate from the colliding nuclei themselves, but from a source that moves at a speed corresponding to one-half of the rapidity of the incident nucleus that is a common center of momentum frame of reference source formed when both nuclei collide, that is the hot quark-gluon plasma fireball.
A very similar enhancement was obtained by the STAR
experiment at the RHIC
. Here results obtained when two colliding systems at 100 A GeV in each beam are considered: in red the heavier Gold-Gold collisions and in blue the smaller Copper-Copper collisions. The energy at RHIC is 11 times greater in the CM frame of reference compared to the earlier CERN work. The important result is that enhancement observed by STAR also increases with the number of participating nucleons. We further note that for the most peripheral events at the smallest number of participants, copper and gold systems show at the same number of participants the same enhancement as could be expected.
Another remarkable feature of these results comparing CERN and STAR is that the enhancement is of similar magnitude for the vastly different energy available in the reaction. This near energy independence of the enhancement also agrees with the quark-gluon plasma approach regarding the mechanism of production of these particles and confirms that a quark-gluon plasma is created over a wide range of collision energies, very probably once a minimal energy threshold is exceeded.
yield ratio. A possible structure has been predicted, and indeed, an unexpected structure is seen in the ratio of particles comprising the positive kaon K (comprising anti s-quarks and up-quark) and positive pion
particles, seen in the figure (solid symbols). The rise and fall (square symbols) of the ratio has been reported by the CERN NA49
. The reason the negative kaon particles do not show this "horn" feature is that the s-quarks prefer to hadronize bound in the Lambda particle, where the counterpart structure is observed. The first exploratory data point from BNL-RHIC-STAR
(red star) in figure agrees with the CERN data.
In view of these results the objective of ongoing NA61/SHINE
experiment at CERN SPS
and the proposed low energy run at BNL RHIC where in particular the STAR detector
can search for the onset of production of quark-gluon plasma as function of energy in the domain where the horn maximum is seen, in order to improve the understanding of these results, and to record the behavior of other related quark-gluon plasma observables.
and charm
are of particular interest. The coincident high yield of charm and strangeness present at LHC will lead to copious production of . Other heavy flavor particles, some which have not been even discovered at this time are also likely to appear. In that way the Strangeness has turned today more generally into Quark Flavor signature of quark-gluon plasma.
Quark-gluon plasma
A quark–gluon plasma or quark soup is a phase of quantum chromodynamics which exists at extremely high temperature and/or density. This phase consists of asymptotically free quarks and gluons, which are several of the basic building blocks of matter...
(or QGP) formation and properties. Unlike up
Up quark
The up quark or u quark is the lightest of all quarks, a type of elementary particle, and a major constituent of matter. It, along with the down quark, forms the neutrons and protons of atomic nuclei...
and down quark
Down quark
The down quark or d quark is the second-lightest of all quarks, a type of elementary particle, and a major constituent of matter. It, along with the up quark, forms the neutrons and protons of atomic nuclei...
s, from which everyday matter is made, strange quark
Strange quark
The strange quark or s quark is the third-lightest of all quarks, a type of elementary particle. Strange quarks are found in hadrons, which are subatomic particles. Example of hadrons containing strange quarks include kaons , strange D mesons , Sigma baryons , and other strange particles...
s are formed in pair production
Pair production
Pair production refers to the creation of an elementary particle and its antiparticle, usually from a photon . For example an electron and its antiparticle, the positron, may be created...
processes in collisions between constituents of the plasma. The dominant mechanism of production involves gluon
Gluon
Gluons are elementary particles which act as the exchange particles for the color force between quarks, analogous to the exchange of photons in the electromagnetic force between two charged particles....
s only present when matter has changed into the QGP phase. When QGP disassembles into hadron
Hadron
In particle physics, a hadron is a composite particle made of quarks held together by the strong force...
s in a breakup process, the high availability of strange antiquarks
Quark
A quark is an elementary particle and a fundamental constituent of matter. Quarks combine to form composite particles called hadrons, the most stable of which are protons and neutrons, the components of atomic nuclei. Due to a phenomenon known as color confinement, quarks are never directly...
helps to produce antimatter containing multiple strange quarks, which is otherwise rarely made. Similar considerations are at present made for the heavier charm quark
Charm quark
The charm quark or c quark is the third most massive of all quarks, a type of elementary particle. Charm quarks are found in hadrons, which are subatomic particles made of quarks...
flavor which is made earlier on at the beginning of the collision process in first interactions and is only abundant in the high-energy environments that will be provided by CERN
CERN
The European Organization for Nuclear Research , known as CERN , is an international organization whose purpose is to operate the world's largest particle physics laboratory, which is situated in the northwest suburbs of Geneva on the Franco–Swiss border...
's Large Hadron Collider
Large Hadron Collider
The Large Hadron Collider is the world's largest and highest-energy particle accelerator. It is expected to address some of the most fundamental questions of physics, advancing the understanding of the deepest laws of nature....
.
Matter formation in the early universe
The majority of matterMatter
Matter is a general term for the substance of which all physical objects consist. Typically, matter includes atoms and other particles which have mass. A common way of defining matter is as anything that has mass and occupies volume...
in the universe
Universe
The Universe is commonly defined as the totality of everything that exists, including all matter and energy, the planets, stars, galaxies, and the contents of intergalactic space. Definitions and usage vary and similar terms include the cosmos, the world and nature...
is found in atomic nuclei
Atomic nucleus
The nucleus is the very dense region consisting of protons and neutrons at the center of an atom. It was discovered in 1911, as a result of Ernest Rutherford's interpretation of the famous 1909 Rutherford experiment performed by Hans Geiger and Ernest Marsden, under the direction of Rutherford. The...
, which are made of neutron
Neutron
The neutron is a subatomic hadron particle which has the symbol or , no net electric charge and a mass slightly larger than that of a proton. With the exception of hydrogen, nuclei of atoms consist of protons and neutrons, which are therefore collectively referred to as nucleons. The number of...
s and proton
Proton
The proton is a subatomic particle with the symbol or and a positive electric charge of 1 elementary charge. One or more protons are present in the nucleus of each atom, along with neutrons. The number of protons in each atom is its atomic number....
s. These neutrons and protons are made up of smaller particles called quarks. For every type of matter particle
Particle physics
Particle physics is a branch of physics that studies the existence and interactions of particles that are the constituents of what is usually referred to as matter or radiation. In current understanding, particles are excitations of quantum fields and interact following their dynamics...
there is a corresponding antiparticle
Antiparticle
Corresponding to most kinds of particles, there is an associated antiparticle with the same mass and opposite electric charge. For example, the antiparticle of the electron is the positively charged antielectron, or positron, which is produced naturally in certain types of radioactive decay.The...
with the same mass and the opposite charge. It is hypothesized that during the first few instants of the universe
Big Bang
The Big Bang theory is the prevailing cosmological model that explains the early development of the Universe. According to the Big Bang theory, the Universe was once in an extremely hot and dense state which expanded rapidly. This rapid expansion caused the young Universe to cool and resulted in...
, it was composed of almost equal amounts of matter and antimatter, and thus contained nearly equal number of quarks and antiquarks. Once the Universe expanded and cooled to a critical temperature
Critical point (thermodynamics)
In physical chemistry, thermodynamics, chemistry and condensed matter physics, a critical point, also called a critical state, specifies the conditions at which a phase boundary ceases to exist...
of approximately , quarks combined into normal matter and antimatter. Antimatter annihilated
Annihilation
Annihilation is defined as "total destruction" or "complete obliteration" of an object; having its root in the Latin nihil . A literal translation is "to make into nothing"....
with matter up to the small initial asymmetry of about one part in five billion, leaving the matter around us. Free and separate individual quarks and antiquarks have never been observed in experiments—quarks and antiquarks are always found in groups of three (baryon
Baryon
A baryon is a composite particle made up of three quarks . Baryons and mesons belong to the hadron family, which are the quark-based particles...
s), or bound in quark–antiquark pairs (meson
Meson
In particle physics, mesons are subatomic particles composed of one quark and one antiquark, bound together by the strong interaction. Because mesons are composed of sub-particles, they have a physical size, with a radius roughly one femtometer: 10−15 m, which is about the size of a proton...
s).
Quark–gluon plasma in the early universe and in the laboratory
Free quarks probably existed in the extreme conditions of the very early universe until about 30 microsecondMicrosecond
A microsecond is an SI unit of time equal to one millionth of a second. Its symbol is µs.A microsecond is equal to 1000 nanoseconds or 1/1000 millisecond...
s after the Big Bang, in a very hot gas
Gas
Gas is one of the three classical states of matter . Near absolute zero, a substance exists as a solid. As heat is added to this substance it melts into a liquid at its melting point , boils into a gas at its boiling point, and if heated high enough would enter a plasma state in which the electrons...
of free quarks, antiquarks and gluon
Gluon
Gluons are elementary particles which act as the exchange particles for the color force between quarks, analogous to the exchange of photons in the electromagnetic force between two charged particles....
s. This gas is called a quark–gluon plasma (QGP), since the quark-interaction charge (color charge
Color charge
In particle physics, color charge is a property of quarks and gluons that is related to the particles' strong interactions in the theory of quantum chromodynamics . Color charge has analogies with the notion of electric charge of particles, but because of the mathematical complications of QCD,...
) is mobile and quarks and gluons move around. This is possible because at a high temperature the early universe is in a different vacuum state, in which normal matter can not exist but quarks and gluons can, they are deconfined
Deconfinement
In physics, deconfinement is the property of a phase in which certain particles are allowed to exist as free excitations, rather than only within bound states...
. In order to recreate this deconfined
Deconfinement
In physics, deconfinement is the property of a phase in which certain particles are allowed to exist as free excitations, rather than only within bound states...
phase of matter
Phase (matter)
In the physical sciences, a phase is a region of space , throughout which all physical properties of a material are essentially uniform. Examples of physical properties include density, index of refraction, and chemical composition...
in the laboratory it is necessary to exceed a minimum temperature or, equivalently, energy density
Energy density
Energy density is a term used for the amount of energy stored in a given system or region of space per unit volume. Often only the useful or extractable energy is quantified, which is to say that chemically inaccessible energy such as rest mass energy is ignored...
. Nuclear collisions
Relativistic nuclear collisions
When atomic nuclei, generally called heavy-ions,collide at very high energies, such that thekinetic energy exceeds significantly the rest mass energy,many strongly interacting particles are produced...
at relativistic energies are the experimental tool that has been developed in the past 30 years to form, study, and explore quark–gluon plasma in laboratory. In a small space domain we thus create for a very short time conditions akin in its properties to the early Universe at the age of 10–40 microseconds. Discovery of this new QGP state of matter
State of matter
States of matter are the distinct forms that different phases of matter take on. Solid, liquid and gas are the most common states of matter on Earth. However, much of the baryonic matter of the universe is in the form of hot plasma, both as rarefied interstellar medium and as dense...
has been announced both at CERN
CERN
The European Organization for Nuclear Research , known as CERN , is an international organization whose purpose is to operate the world's largest particle physics laboratory, which is situated in the northwest suburbs of Geneva on the Franco–Swiss border...
and at Brookhaven National Laboratory
Brookhaven National Laboratory
Brookhaven National Laboratory , is a United States national laboratory located in Upton, New York on Long Island, and was formally established in 1947 at the site of Camp Upton, a former U.S. Army base...
(BNL). At this time comprehensive experimental evidence about its properties is being assembled.
The process of the formation of quark–gluon plasma lasts little longer than the time the light takes to pass through the volume occupied by the atomic nucleus used to produce the ultra-high pressure and temperature in the highly-energetic collision. After this brief time the hot drop of quark plasma evaporates in a process called hadronization
Hadronization
In particle physics, hadronization is the process of the formation of hadrons out of quarks and gluons. This occurs after high-energy collisions in a particle collider in which free quarks or gluons are created. Due to postulated colour confinement, these cannot exist individually...
. The short duration of the laboratory's collision illustrated above makes the study of free quarks in quark–gluon plasma an experimental challenge.
Strangeness in quark-gluon plasma
The diagnosis and the study of the properties of quark-gluon plasma can be undertaken using quarks not present in matter seen around us. The experimental and theoretical work relies on the idea of strangeness enhancement. This was the first observable of quark-gluon plasma proposed in 1980 by RafelskiJohann Rafelski
Johann Rafelski is a German-American theoretical physicist and author. He is Professor of Physics at The University of Arizona in Tucson, guest scientist at CERN , and has been LMU-Excellent Guest Professor at the Ludwig Maximilian University of Munich in Munich, Germany.Rafelski’s current...
and Hagedorn
Rolf Hagedorn
Rolf Hagedorn was a German theoretical physicists working at CERN. He is known for the idea that hadronic matter has a "melting point". The Hagedorn temperature is named in his honor.-Early life:...
. Unlike the up and down quarks, strange quarks are not brought into the reaction by the colliding nuclei. Therefore, any strange quarks or antiquarks observed in experiments have been "freshly" made from the kinetic energy of colliding nuclei. Conveniently, the mass
Mass
Mass can be defined as a quantitive measure of the resistance an object has to change in its velocity.In physics, mass commonly refers to any of the following three properties of matter, which have been shown experimentally to be equivalent:...
of strange quarks and antiquarks is equivalent to the temperature or energy at which protons, neutrons and other hadron
Hadron
In particle physics, a hadron is a composite particle made of quarks held together by the strong force...
s dissolve into quarks. This means that the abundance of strange quarks is sensitive to the conditions, structure and dynamics of the deconfined matter phase, and if their number is large it can be assumed that deconfinement conditions were reached.
Production of strangeness in the thermal collision process
One cannot assume that under all conditions the yield of strange quarks is in thermal equilibrium. In general, the quark-flavor composition of the plasma varies during its ultra short lifetime as new flavors of quarks such as strangeness are cooked up inside. The up and down quarks from which normal matter is made are easily produced as quark-antiquark pairs in the hot fireball because they have small masses. On the other hand the next lightest quark flavor, strange quarks, will reach its high quark-gluon plasma thermal abundance only on the most violent collisions generating high temperatures and that at the end of the cooking process.Gluon fusion into strangeness
This is only possible due to a new process, the gluon fusion, as shown by Rafelski and Müller in 1981. The top section of the figure shows gluon fusion in form of the intuitively understood Feynman diagramFeynman diagram
Feynman diagrams are a pictorial representation scheme for the mathematical expressions governing the behavior of subatomic particles, first developed by the Nobel Prize-winning American physicist Richard Feynman, and first introduced in 1948...
s: gluons are the wavy lines; strange quarks are the solid lines; time runs from left to right. The bottom section is the process where the heavier quark pair arises from the lighter pair of quarks shown as dashed lines. The gluon fusion process occurs almost ten times faster than the quark based strangeness process, and allows achievement of the high thermal yield where the quark based process would fail to do so during the duration of the "micro-bang". The gluon collisions here are occurring within the thermal matter phase and are thus different from the high energy processes that can ensue in the early stages of the collisions when the nuclei crash into each other. The heavier, charm and bottom quarks are produced there dominantly. The study in relativistic nuclear (heavy ion) collisions of charmed and soon also bottom hadronic particle production beside strangeness will provide complementary and important confirmation of the mechanisms of formation, evolution and hadronization of quark gluon plasma.
Strangeness (and charm) hadronization
These newly cooked strange quarks find their way into a multitude of different final particles that emerge as the hot quark-gluon plasma fireball breaks up, see the scheme of different processes in figure. Given the ready supply of antiquarks in the "fireball", one also finds a multitude of antimatter particles containing more than one strange quark. On the other hand, in a system involving a cascade of nucleon-nucleon collisions, multi-strange antimatter are produced less frequently considering that several relatively improbable events must occur in the same collision process. For this reason one expects that the yield of multi-strange antimatter particles produced in the presence of quark matter is enhanced compared to conventional series of reactions.Strange quarks also bind with the heavier charm and bottom quarks which also like to bind with each other. Thus in presence of a large number of these quarks quite unusually abundant exotic particles can be produced, some of these have never been observed before. This should be the case in the forthcoming exploration at the new Large Hadron Collider
Large Hadron Collider
The Large Hadron Collider is the world's largest and highest-energy particle accelerator. It is expected to address some of the most fundamental questions of physics, advancing the understanding of the deepest laws of nature....
at CERN of the particles that have both charm and strange quarks, and even bottom quarks as components.
Strange hadron decay and observation
Strange quarks are naturally radioactiveRadioactive decay
Radioactive decay is the process by which an atomic nucleus of an unstable atom loses energy by emitting ionizing particles . The emission is spontaneous, in that the atom decays without any physical interaction with another particle from outside the atom...
and decay by weak interaction
Weak interaction
Weak interaction , is one of the four fundamental forces of nature, alongside the strong nuclear force, electromagnetism, and gravity. It is responsible for the radioactive decay of subatomic particles and initiates the process known as hydrogen fusion in stars...
s into lighter quarks on a timescale that is extremely long compared with the nuclear-collision times. This makes it relatively easy to detect strange particles through the tracks left by their decay products. Consider as example the decay of a negatively charged Xi baryon (green in figure, dss), into a negative pion
Pion
In particle physics, a pion is any of three subatomic particles: , , and . Pions are the lightest mesons and they play an important role in explaining the low-energy properties of the strong nuclear force....
(d) and a neutral Lambda baryon (uds). Subsequently, the Lambda decays into a proton and another negative pion. In general this is the signature of the decay of a Xi. Although the negative Omega baryon (sss) has a similar final state decay topology, it can be clearly distinguished from the Xi because its decay products are different.
Measurement of abundant formation of Xi (uss/dss), Omega (sss) and especially their antiparticles is an important cornerstone of the claim that quark-gluon plasma has been formed. This abundant formation is often presented in comparison with the scaled expectation from normal proton-proton collisions; however, such a comparison is not a necessary step in view of the large absolute yields which defy conventional model expectations. The overall yield of strangeness is also larger than expected if the new form of matter has been achieved. However, considering that the light quarks are also produced in gluon fusion processes, one expects increased production of all hadron
Hadron
In particle physics, a hadron is a composite particle made of quarks held together by the strong force...
s. The study of the relative yields of strange and non strange particles provides information about the competition of these processes and thus the reaction mechanism of particle production.
S-S collisions at SPS-CERN with projectile energy 200 A GeV on fixed target
The first strangeness signature of a possible quark-gluon formation was presented in May 1990 by the CERN-NA35 experimental collaboration at the Quark Matter meeting in MentonMenton
Menton is a commune in the Alpes-Maritimes department in the Provence-Alpes-Côte d'Azur region in southeastern France.Situated on the French Riviera, along the Franco-Italian border, it is nicknamed la perle de la France ....
, France
France
The French Republic , The French Republic , The French Republic , (commonly known as France , is a unitary semi-presidential republic in Western Europe with several overseas territories and islands located on other continents and in the Indian, Pacific, and Atlantic oceans. Metropolitan France...
These results on Antilambda formation in S-S reaction shown in the figure indicate a significant enhancement of the production of this antimatter particle comprising one antistrange quark as well as antiup and antidown quarks. All three constituents of the Lambda particle are newly produced in the reaction. The expected no-quark-gluon plasma production yield is shown at the bottom of the figure. These results are presented as function of the variable called rapidity
Rapidity
In relativity, rapidity is an alternative to speed as a framework for measuring motion. On parallel velocities rapidities are simply additive, unlike speeds at relativistic velocities. For low speeds, rapidity and speed are proportional, but for high speeds, rapidity takes a larger value. The...
which characterizes the speed of the source. The peak of emission indicates that the additionally formed antimatter particles do not originate from the colliding nuclei themselves, but from a source that moves at a speed corresponding to one-half of the rapidity of the incident nucleus that is a common center of momentum frame of reference source formed when both nuclei collide, that is the hot quark-gluon plasma fireball.
Systematics of strange matter and antimatter creation
The work of Koch, Muller, Rafelski predicts that in a quark-gluon plasma hadronization process the enhancement for each particle species increases with the strangeness content of the particle. The enhancements for particles carrying one, two and three strange or antistrange quarks were measured and this effect was demonstrated by the CERN WA97 experiment in time for the CERN announcement in 2000 of a possible quark-gluon plasma formation in its experiments. These results were elaborated by the successor collaboration NA57 as shown in figure. The gradual rise of the enhancement as a function of the variable representing the amount of nuclear matter participating in the collisions, and thus as function of the geometric centrality of nuclear collision strongly favors the quark-gluon plasma source over normal matter reactions.A very similar enhancement was obtained by the STAR
STAR detector
The STAR detector is one of the four experiments at the Relativistic Heavy Ion Collider in Brookhaven National Laboratory, United States....
experiment at the RHIC
Relativistic Heavy Ion Collider
The Relativistic Heavy Ion Collider is one of two existing heavy-ion colliders, and the only spin-polarized proton collider in the world. It is located at Brookhaven National Laboratory in Upton, New York and operated by an international team of researchers...
. Here results obtained when two colliding systems at 100 A GeV in each beam are considered: in red the heavier Gold-Gold collisions and in blue the smaller Copper-Copper collisions. The energy at RHIC is 11 times greater in the CM frame of reference compared to the earlier CERN work. The important result is that enhancement observed by STAR also increases with the number of participating nucleons. We further note that for the most peripheral events at the smallest number of participants, copper and gold systems show at the same number of participants the same enhancement as could be expected.
Another remarkable feature of these results comparing CERN and STAR is that the enhancement is of similar magnitude for the vastly different energy available in the reaction. This near energy independence of the enhancement also agrees with the quark-gluon plasma approach regarding the mechanism of production of these particles and confirms that a quark-gluon plasma is created over a wide range of collision energies, very probably once a minimal energy threshold is exceeded.
The horn in K-to-pi ratio and the onset of deconfinement
One of most interesting questions is if there is a threshold in reaction energy and/or volume size which needs to be exceeded in order to form a domain in which quarks can move freely. It is natural to expect that if such a threshold exists the particle yields/ratios we have shown above should indicate that. One of the most accessible signatures would be the relative KaonKaon
In particle physics, a kaon is any one of a group of four mesons distinguished by the fact that they carry a quantum number called strangeness...
yield ratio. A possible structure has been predicted, and indeed, an unexpected structure is seen in the ratio of particles comprising the positive kaon K (comprising anti s-quarks and up-quark) and positive pion
Pion
In particle physics, a pion is any of three subatomic particles: , , and . Pions are the lightest mesons and they play an important role in explaining the low-energy properties of the strong nuclear force....
particles, seen in the figure (solid symbols). The rise and fall (square symbols) of the ratio has been reported by the CERN NA49
NA49 experiment
The NA49 experiment was a particle physics experiment that took place in the North Area of the Super Proton Synchrotron at CERN. It used a large-acceptance hadron detector to investigate reactions induced by the collision of various heavy ions on targets made of a variety of elements...
. The reason the negative kaon particles do not show this "horn" feature is that the s-quarks prefer to hadronize bound in the Lambda particle, where the counterpart structure is observed. The first exploratory data point from BNL-RHIC-STAR
STAR detector
The STAR detector is one of the four experiments at the Relativistic Heavy Ion Collider in Brookhaven National Laboratory, United States....
(red star) in figure agrees with the CERN data.
In view of these results the objective of ongoing NA61/SHINE
NA61/SHINE
NA61/SHINE is a particle physics experiment at the Super Proton Synchrotron at the European Organization for Nuclear Research...
experiment at CERN SPS
Super Proton Synchrotron
The Super Proton Synchrotron is a particle accelerator of the synchrotron type at CERN. It is housed in a circular tunnel, in circumference, straddling the border of France and Switzerland near Geneva, Switzerland. The SPS was designed by a team led by John Adams, director-general of what was...
and the proposed low energy run at BNL RHIC where in particular the STAR detector
STAR detector
The STAR detector is one of the four experiments at the Relativistic Heavy Ion Collider in Brookhaven National Laboratory, United States....
can search for the onset of production of quark-gluon plasma as function of energy in the domain where the horn maximum is seen, in order to improve the understanding of these results, and to record the behavior of other related quark-gluon plasma observables.
Strangeness and Flavor Signatures of QGP at the LHC
At the much higher energy of the LHC the production of strangeness in quark gluon plasma saturates leading to quark-level chemical equilibrium yield. As the fireball of matter expands and breaks apart this in turn provides a very high abundance of strange hadrons. Among these, heavy mesons comprising strange quarks, and a heavy quark, such as bottomBottomness
In physics, bottomness also called beauty, is a flavour quantum number reflecting the difference between the number of bottom antiquarks and the number of bottom quarks that are present in a particle: B^\prime = -Bottom quarks have a bottomness of −1 while bottom antiquarks have a...
and charm
Charm quark
The charm quark or c quark is the third most massive of all quarks, a type of elementary particle. Charm quarks are found in hadrons, which are subatomic particles made of quarks...
are of particular interest. The coincident high yield of charm and strangeness present at LHC will lead to copious production of . Other heavy flavor particles, some which have not been even discovered at this time are also likely to appear. In that way the Strangeness has turned today more generally into Quark Flavor signature of quark-gluon plasma.
Outlook
The strangeness production and its diagnostic potential as signature of quark-gluon plasma has been discussed for nearly 30 years. The work in this field today focuses on the theoretical interpretation of the overall particle production data and the derivation of the resulting properties of the bulk of quark-gluon plasma at the time of breakup. The global description of all produced particles can be attempted based on the picture of hadronizing hot drop of quark-gluon plasma or, alternatively, on the picture of confined and equilibrated hadron matter. In both cases one describes the data within the statistical thermal production model, but considerable differences in detail differentiate the nature of the source of these particles. The experimental groups working in the field also like to develop their own analysis models and the outside observer sees many different analysis results. For this reason the presentation of the experimental results was made above without a comparison of data to model, so that the results following the pattern predicted can speak for themselves. There are as many as 10 different particles species which follow the pattern predicted for the QGP as function of reaction energy, reaction centrality, and strangeness content. At yet higher LHC energies saturation of strangeness yield and binding to heavy flavor open new experimental opportunities.See also
- QGP
- Quark matter
- HadronizationHadronizationIn particle physics, hadronization is the process of the formation of hadrons out of quarks and gluons. This occurs after high-energy collisions in a particle collider in which free quarks or gluons are created. Due to postulated colour confinement, these cannot exist individually...
- StrangeletStrangeletA strangelet is a hypothetical particle consisting of a bound state of roughly equal numbers of up, down, and strange quarks. Its size would be a minimum of a few femtometres across . Once the size becomes macroscopic , such an object is usually called a quark star or "strange star" rather than a...